Teachable Moments | September 30, 2021
Learn About the Universe With the James Webb Space Telescope
Get a look into the science and engineering behind the largest and most powerful space telescope ever built while exploring ways to engage learners in the mission.
NASA is launching the largest, most powerful space telescope ever. The James Webb Space Telescope will look back at some of the earliest stages of the universe, gather views of early star and galaxy formation, and provide insights into the formation of planetary systems, including our own solar system.
Read on to learn more about what the space-based observatory will do, how it works, and how to engage learners in the science and engineering behind the mission.
What It Will Do
The James Webb Space Telescope, or JWST, was developed through a partnership between NASA and the European and Canadian space agencies. It will build upon and extend the discoveries made by the Hubble Space Telescope to help unravel mysteries of the universe. First, let's delve into what scientists hope to learn with the Webb telescope.
A look at the James Webb Space Telescope, its mission and the incredible technological challenge this mission presents. | Watch on YouTube
How Galaxies Evolve
What the first galaxies looked like and when they formed is not known, and the Webb telescope is designed to help scientists learn more about that early period of the universe. To better understand what the Webb telescope will study, it’s helpful to know what happened in the early universe, before the first stars formed.
The universe, time, and space all began about 13.8 billion years ago with the Big Bang. For the first few hundred-thousand years, the universe was a hot, dense flood of protons, electrons, and neutrons, the tiny particles that make up atoms. As the universe cooled, protons and neutrons combined into ionized hydrogen and helium, which had a positive charge, and eventually attracted all those negatively charged electrons. This process, known as recombination, occurred about 240,000 to 300,000 years after the Big Bang.

This image shows the temperature fluctuations (shown as color differences) in the cosmic microwave background from a time when the universe was less than 400,000 years old. The image was captured by the Wilkinson Microwave Anisotropy Probe, or WMAP, which spent nine years, from 2001 to 2010, collecting data on the early universe. Credit: NASA | › Full image and caption | + Expand image
Light that previously couldn’t travel without being scattered by the dense ionized plasma of early particles could now travel freely. The very first form of light we can look back and see comes from this time and is known as the cosmic microwave background radiation. It is essentially a map of temperature fluctuations across the universe left behind from the Big Bang. The fluxuations give clues about the origin of galaxies and the large-scale structure of galaxies. There were still no stars in the universe at this time, so the next several hundred million years are known as the cosmic dark ages.
Current theory predicts that the earliest stars were big – 30 to 300 times the size of our Sun – and burned quickly, ending in supernova explosions after just a few million years. (For comparison, our Sun has a lifespan of about 10 billion years and will not go supernova.) Observing these luminous supernovae is one of the few ways scientists could study the earliest stars. That is vital to understanding the formation of objects such as the first galaxies.
By using the Webb telescope to compare the earliest galaxies with those of today, scientists hope to understand how they form, what gives them their shape, how chemical elements are distributed across galaxies, how central black holes influence their galaxies, and what happens when galaxies collide.
Learn how the James Webb Space Telescope's ability to look farther into space than ever before will bring newborn galaxies into view. | Watch on YouTube
How Stars and Planetary Systems Form
Stars and their planetary systems form within massive clouds of dust and gas. It's impossible to see into these clouds with visible light, so the Webb telescope is equipped with science instruments that use infrared light to peer into the hearts of stellar nurseries. When viewing these nurseries in the mid-infrared – as the Webb telescope is designed to do – the dust outside the dense star forming regions glows and can be studied directly. This will allow astronomers to observe the details of how stars are born and investigate why most stars form in groups as well as how planetary systems begin and evolve.

This mosaic image is the sharpest wide-angle view ever obtained of the starburst galaxy, Messier 82 (M82). The galaxy is remarkable for its bright blue disk, webs of shredded clouds and fiery-looking plumes of glowing hydrogen blasting out of its central regions.Throughout the galaxy's center, young stars are being born 10 times faster than they are inside our entire Milky Way Galaxy. Credit: NASA, ESA, and The Hubble Heritage Team (STScI/AURA); Acknowledgment: J. Gallagher (University of Wisconsin), M. Mountain (STScI), and P. Puxley (National Science Foundation) | › Full image and caption | + Expand image
How Exoplanets and Our Solar System Evolve
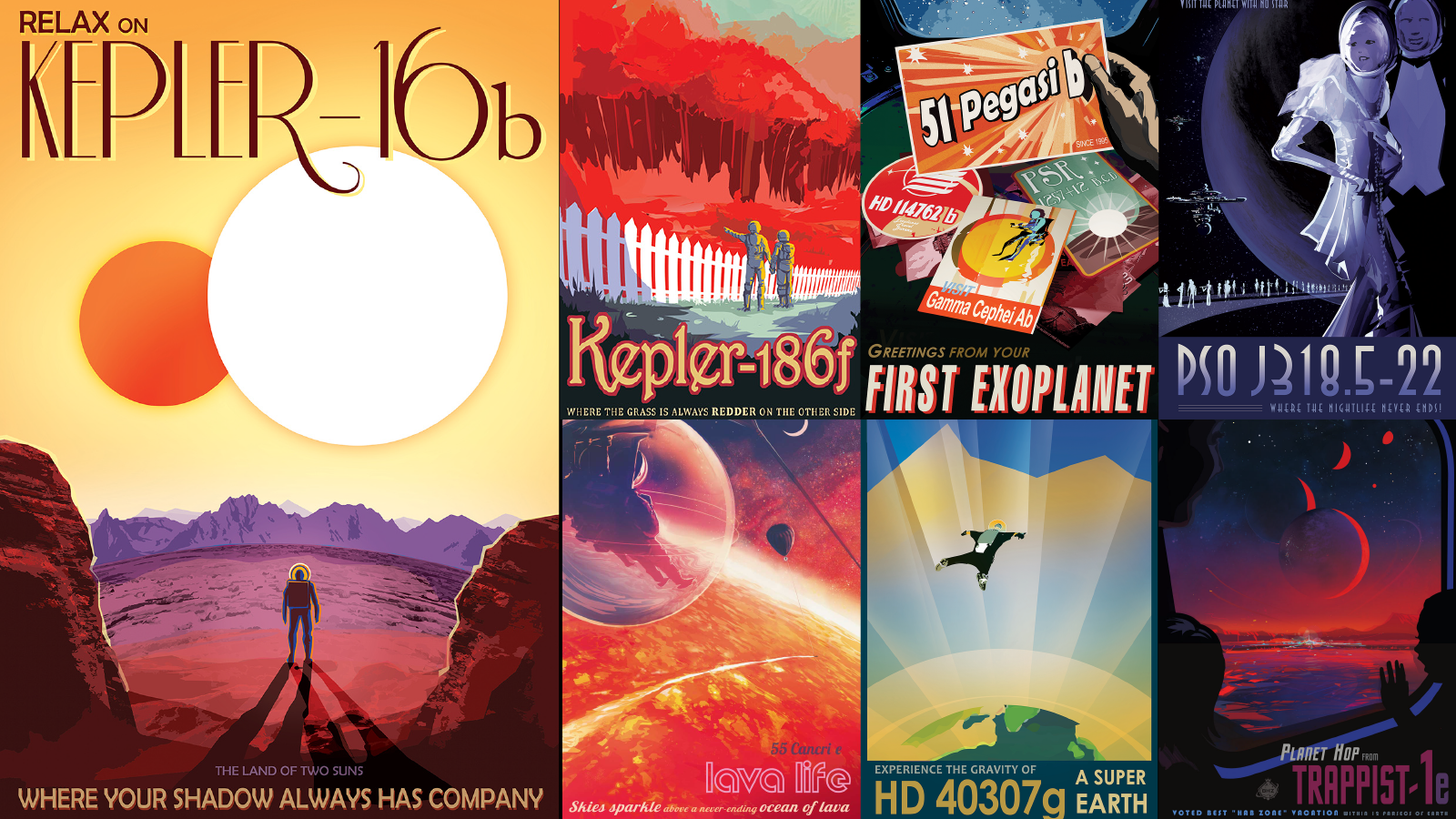
As we make more discoveries about exoplanets, artists at NASA are imagining what future explorers might encounter on these faraway worlds as part of the Exoplanet Travel Bureau poster series. Credit: NASA | › View and download the posters | + Expand image
The first planet outside our solar system, or exoplanet, was discovered in 1992. Since then, scientists have found thousands more exoplanets and estimate that there are hundreds of billions in the Milky Way galaxy alone. There are many waiting to be discovered and there is more to learn about the exoplanets themselves, such as what makes up their atmospheres and what their weather and seasons may be like. The Webb telescope will help scientists do just that.
In our own solar system, the Webb telescope will study planets and other objects to help us learn more about our solar neighborhood. It will be able to complement studies of Mars being carried out by orbiters, landers, and rovers by searching for molecules that may be signs of past or present life. It is powerful enough to identify and characterize icy comets in the far reaches of our solar system. And it can be used to study places like Saturn, Uranus, and Neptune while there are no active missions at those planets.
How It Works
The Webb telescope has unique capabilities enabled by the way it views the universe, its size, and the new technologies aboard. Here's how it works.
Peering Into the Infrared
To see ancient, distant galaxies, the Webb telescope was built with instruments sensitive to light in the near- and mid-infrared wavelengths.
Light leaving these galaxies can take billions of years to reach Earth, so when we see these objects, we’re actually seeing what they looked like in the past. The farther something is from Earth, the farther back in time it is when we observe it. So when we look at light that left objects 13.5 billion years ago, we're seeing what happened in the early universe.
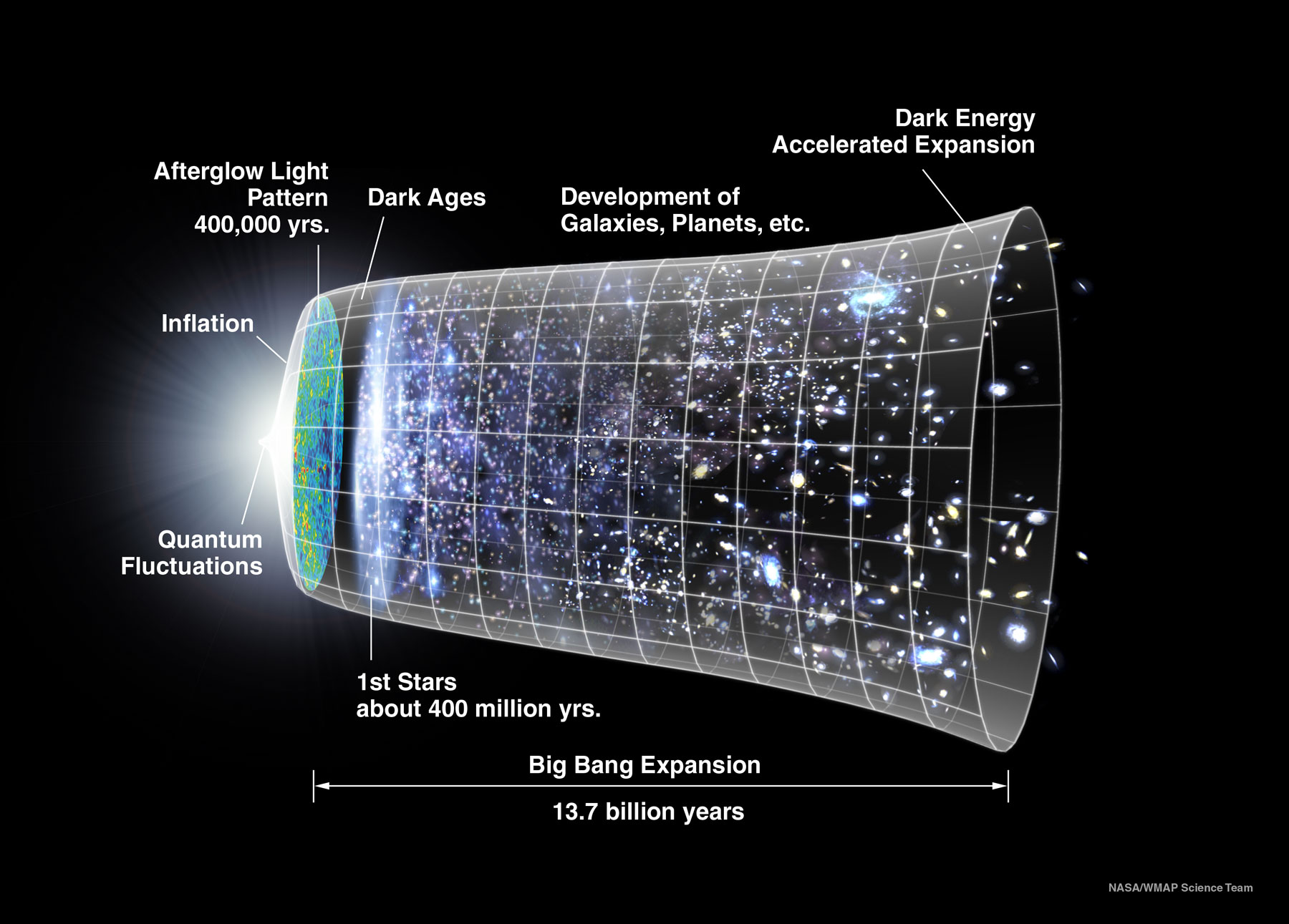
An illustrated timeline of the universe. Credit: WMAP | + Expand image
As light from distant objects travels to Earth, the universe continues to expand, something it’s been doing since the Big Bang. The waves that make up the light get stretched as the universe expands. You can see this effect in action by making an ink mark on a rubber band and observing how the mark stretches out when you pull on the rubber band.

Light waves get stretched as the universe expands similar to how this ink mark stretches out as the elastic is pulled. Get students modeling and exploring this effect with this standards-aligned math lesson. Credit: NASA/JPL-Caltech | + Expand image
What this means for light coming from distant galaxies is that the visible lightwaves you would be able to see with your eyes get stretched out so far that the longer wavelengths shift from visible light into infrared. Scientists refer to this phenomenon as redshift – and the farther away an object is, the more redshift it undergoes.
Webb telescope’s infrared sensing equipment will give scientists the chance to study some of the earliest stars that exploded in supernova events, creating the elements necessary to build planets and form life.
Gathering Light
The first stars were massive, their life cycles ending in supernova explosions. The light from these explosions has traveled so far that it is incredibly dim. This is due to the inverse square law. You experience this effect when a room appears to get darker as you move away from a light source.
To see such dim light, the Webb telescope needs to be extremely sensitive. A telescope’s sensitivity, or its ability to detect faint signals, is related to the size of the mirror it uses to gather light. On the Webb telescope, 18 hexagonal mirrors combine to form a massive primary mirror that is 21 feet (6.5 meters) across.
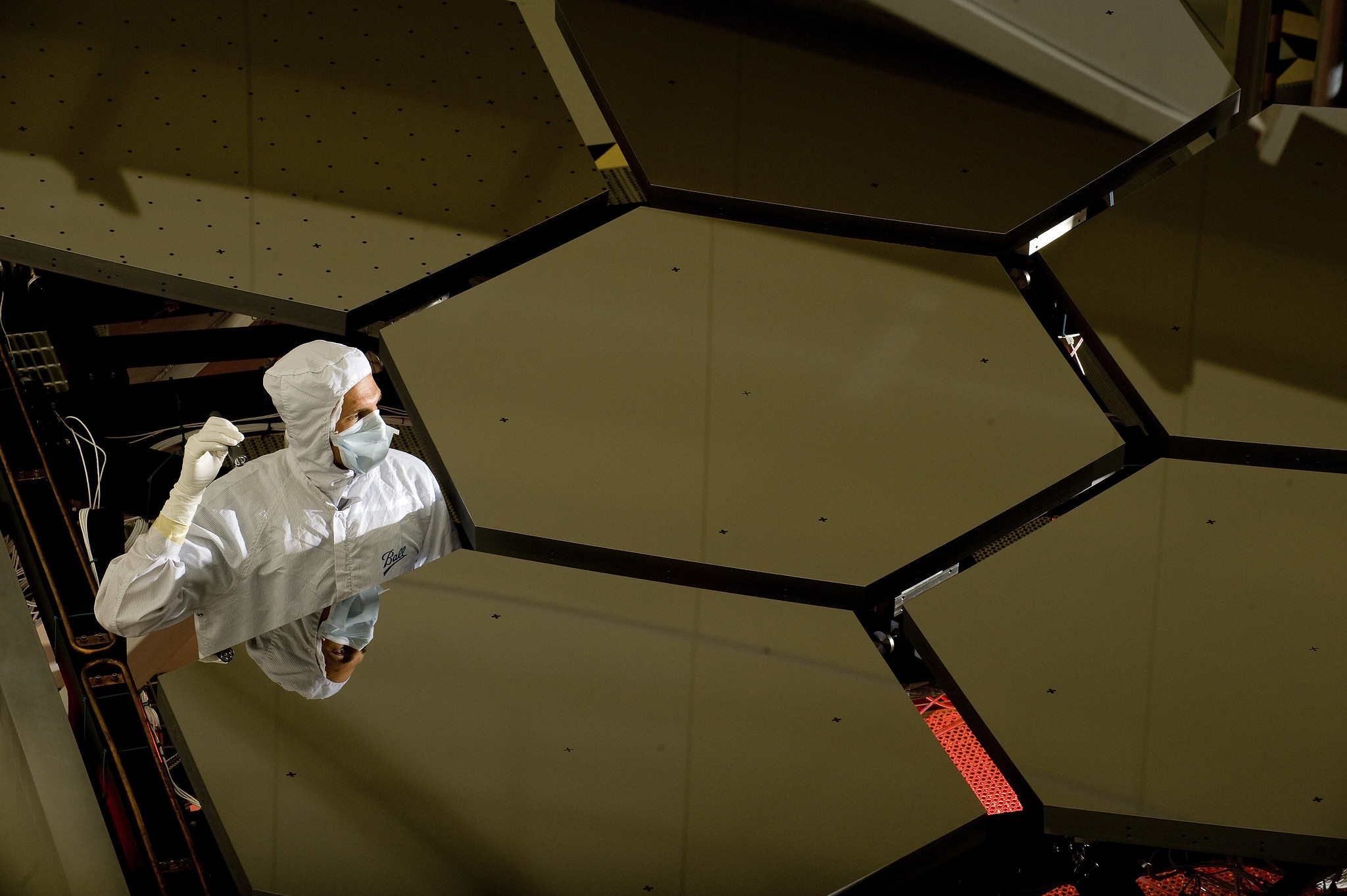
A technician inspects the Webb telescope's honeycomb-shaped mirror. The telescope's primary mirror is 21 feet (6.5 meters) across and is made up of 18 smaller hexagonal mirrors that must fold for launch and unfurl after the telescope reaches its orbit in space. Credit: NASA/MSFC/David Higginbotham/Emmett Given | › Full image and caption | + Expand image
Compared with the Hubble Space Telescope’s eight-foot (2.4 meter) diameter mirror, this gives the Webb telescope more than six times the surface area to collect those distant particles of light known as photons. Hubble’s famous Ultra Deep Field observation captured images of incredibly faint, distant galaxies by pointing at a seemingly empty spot in space for 16 days, but the Webb telescope will be able to make a similar observation in just seven hours.
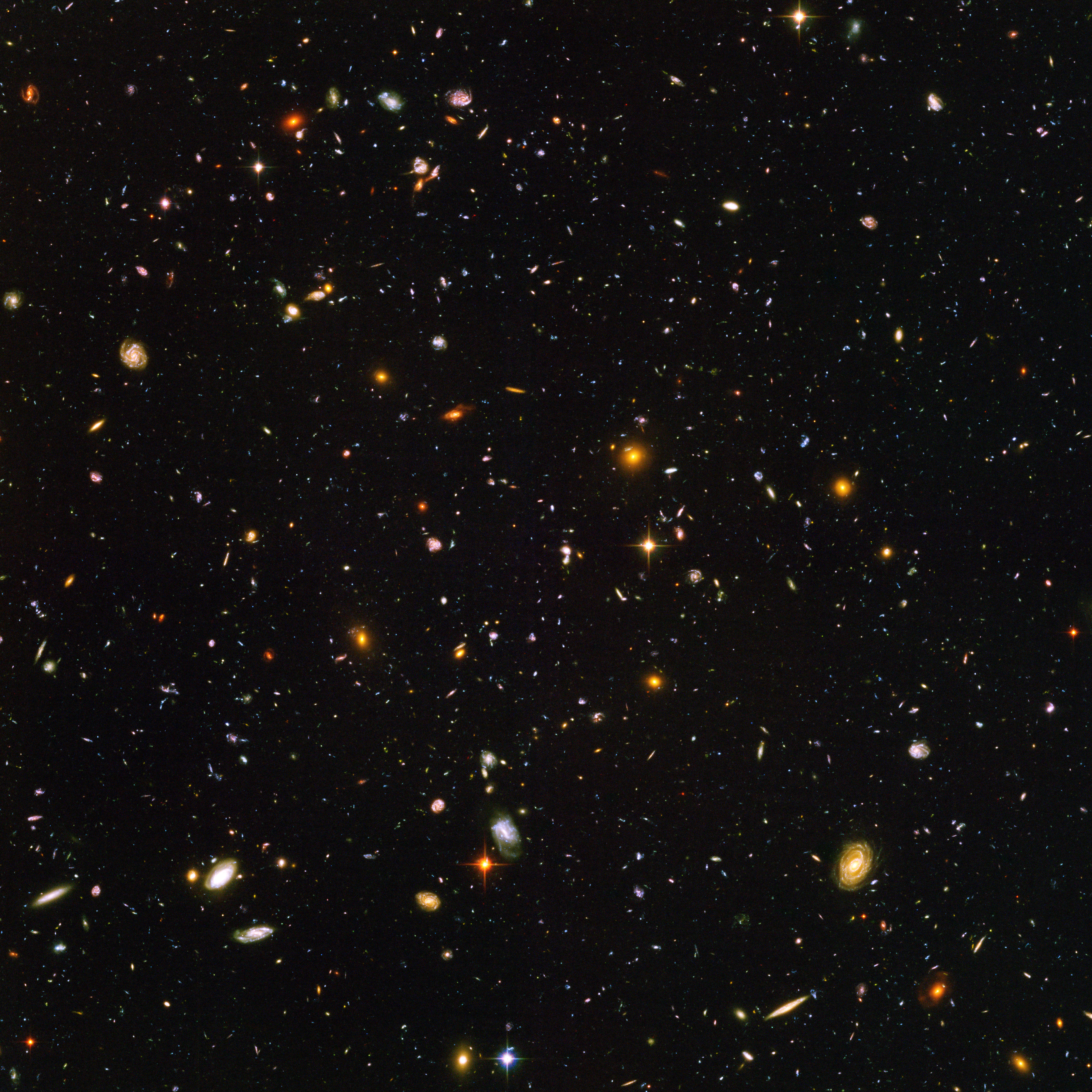
This image, called the Hubble Ultra Deep Field, shows 28 of the more than 500 young galaxies that existed when the universe was less than 1 billion years old. Credit: NASA, ESA, R. Bouwens and G. Illingworth (University of California, Santa Cruz) | › Full image and caption | + Expand image
Keeping Cool
The Webb Telescope gathers its scientific data as infrared light. To detect the faint signals of objects billions of light years away, the instruments inside the telescope have to be kept very cold, otherwise those infrared signals could get lost in the heat of the telescope. Engineers accounted for this with a couple of systems designed to get the instruments cold and keep them cold.
The Webb telescope's orbit around the Sun – sitting about 1 million miles (1.5 million kilometers) from Earth at Lagrange point 2 – keeps the spacecraft pretty far from our planet's heat, but even that’s not enough. To further reduce the temperature on the instruments, the spacecraft will unfurl a tennis-court-size sunshield that will block light and heat from the Sun, Earth, and Moon using five layers of specially coated material. Each layer blocks incoming heat, and the heat that does make it through is redirected out of the sides of the sunshield. Additionally, the vacuum between each layer provides insulation.

The sunshield is made up of five layers of specially coated material designed to block the Webb telescope's sensitive instruments from incoming heat from the Sun, Earth, and Moon. This photo, taken in the cleanroom at Northrop Grumman in Southern California in December 2020, shows the sunshield fully deployed and tensioned as it will be in space. Credit: NASA/Chris Gunn | › Full image and caption | + Expand image
The sunshield is so effective that the temperatures on the Sun-facing side of the telescope could be hot enough to boil water, while on the side closest to the instruments, the temperature could be as low as -394 F (-237 C, 36 K).
That’s cold enough for the near-infrared instruments to operate, but the Mid-Infrared Instrument, or MIRI, needs to be even colder. To bring down the temperature of MIRI, the Webb telescope is equipped with a special cryocooler that pumps chilled helium to the instrument to reduce its operating temperature to about -448 F (-267 C, 6 K).
Spotting Exoplanets
The Webb telescope will search for exoplanets using two different methods.
Using the transit method, the Webb telescope will look for the regular pattern of dimming that occurs when an exoplanet transits its star, or passes between the star and the telescope. The amount of dimming can tell scientists a lot about the passing exoplanet, such as the size of the planet and its distance from the star.

This animation shows how the transit method is used to hunt for planets outside our solar system. When exoplanets transit their parent star, the Webb telescope (like the Kepler space telescope, depicted here) will be able to detect the dip in the star’s brightness, providing scientists with key information about the transiting exoplanet. Students can see this technique in action with this transit math problem. Credit: NASA/JPL-Caltech | + Expand image
The second method the Webb telescope will use to search for exoplanets is direct imaging – capturing actual images of planets beyond our solar system. To enable direct imaging of exoplanets, the Webb telescope is equipped with a coronagraph. Just like you might use your hand to block a bright light, a coronagraph blocks starlight from reaching a telescope’s instruments, allowing a dim exoplanet orbiting a star to be seen.
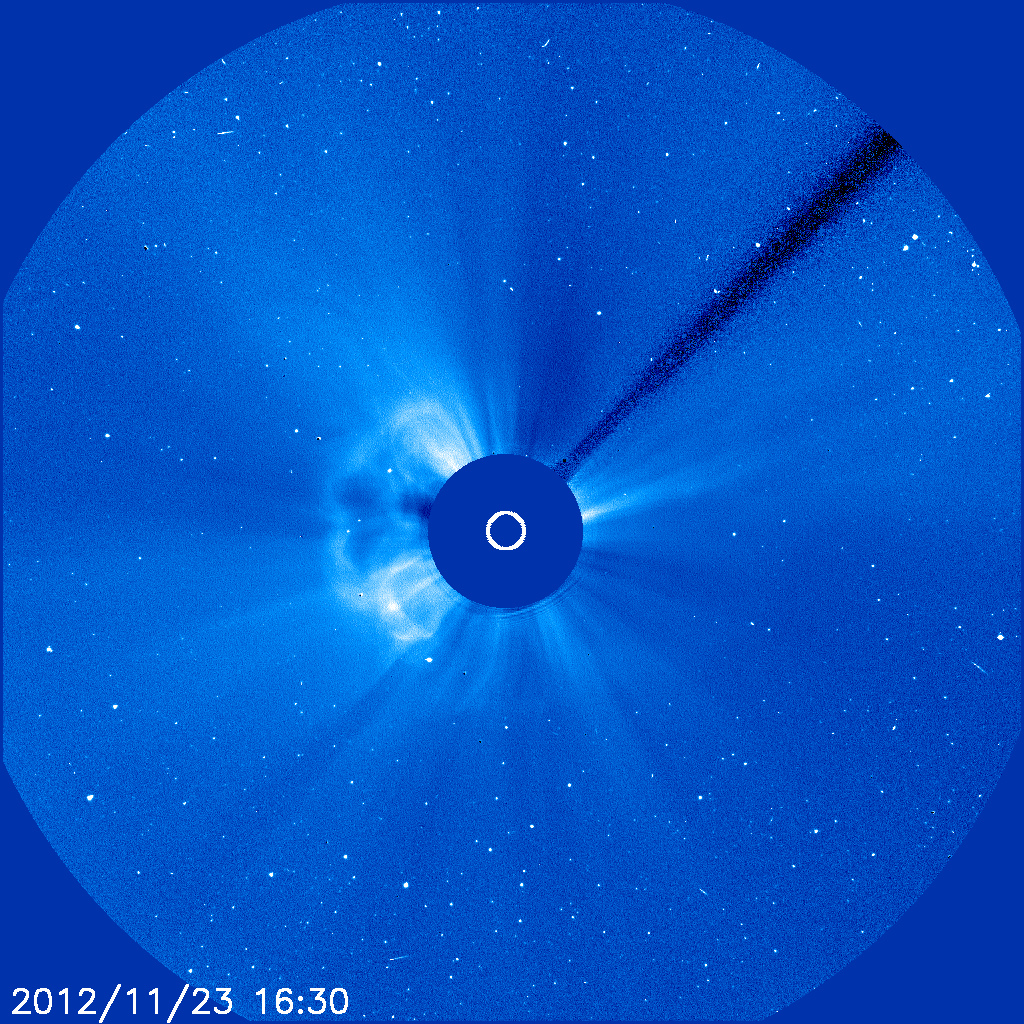
This “coronagraph” image taken by the Solar and Heliospheric Observatory, or SOHO, shows dim features around our Sun. Similarly, direct images of exoplanets captured by the Webb telescope will reveal details normally washed out by the brightness of stars. Credit: ESA&NASA/SOHO | › Full image and caption | + Expand image
The Webb telescope can uncover even more using spectroscopy. Light from a star produces a spectrum, which displays the intensity of light at different wavelengths. When a planet transits its star, some of the light from the star will pass through the planet's atmosphere before reaching the Webb telescope. Since all elements and molecules, such as methane and water, absorb energy at specific wavelengths, spectra from light that has passed through a planet’s atmosphere may contain dark lines known as absorption lines that tell scientists if there are certain elements present.
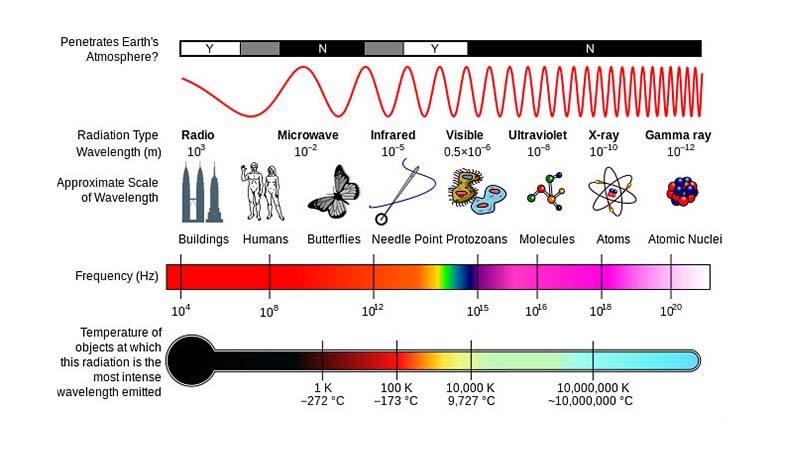
By looking at the unique spectrum produced when the light from a star shines through the atmosphere of a transiting exoplanet, scientists can learn whether certain elements are present on that planet. Credit: NASA | + Expand image
Using direct imaging and spectroscopy, scientists can learn even more about an exoplanet, including its color, seasons, rotation, weather, and vegetation if it exists.
All this could lead scientists to the ultimate exoplanet discovery: an Earth-size planet with an atmosphere like ours in its star’s habitable zone – a place where liquid water could exist.
Setting Up in Space
The Webb telescope will launch from French Guiana on top of an Ariane 5 rocket, a massive rocket capable of lifting the telescope, which weighs nearly 14,000 pounds (6,200 kilograms), to its destination.
The telescope's large mirror and giant sunshield are too big to fit inside the 18-foot (5.4-meter) wide rocket fairing, which protects the spacecraft during launch. To overcome this challenge, engineers designed the telescope's mirror and sunshield to fold for launch.
Two sides of the mirror assembly fold back for launch, allowing them to fit inside the fairing. The sunshield, which is 69.5 feet (21 meters) long and 46.5 feet (14 meters) wide, is carefully folded 12 times like origami so that it's narrow enough for launch. These are just two examples of several folding mechanisms needed to fit the massive telescope in its rocket for launch.
It will take about a month for the Webb telescope to reach its destination and unfurl its mirrors and sunshield. Scientists need another five months to cool down the instruments to their operating temperatures and align the mirrors correctly.
Approximately six months after launch, checkouts should be complete, and the telescope will begin its first science campaign and science operations.
Learn more and follow along with the mission from launch and unfolding to science observations and discovery announcements on the James Webb Space Telescope website.
Teach It
Check out these resources to bring the real-life STEM behind the mission into your teaching with lesson guides for educators, projects and slideshows for students, and more.
Educator Guides
Student Activities
Articles for Students
- What is the James Webb Space Telescope?
- What is the Big Bang?
- What is a galaxy?
- What is a satellite galaxy?
- What is a transit?
- What is a black hole?
- What is a light year?
- What is a nebula?
- What is an exoplanet?
- How many solar systems are in our galaxy?
- How old are galaxies?
- What is a supernova?
- Explore the electromagnetic spectrum
Videos for Students
- Space Place in a Snap: The Solar System’s Formation
- Space Place in a Snap: Searching for Other Planets Like Ours
Resources for Educators and Parents
Events
Explore More
- Mission Website: James Webb Space Telescope
- Photos: James Webb Space Telescope
- Videos: James Webb Space Telescope
- Facts & Figures: Mid-Infrared Instrument (MIRI)
NASA's Universe of Learning materials are based upon work supported by NASA under award number NNX16AC65A to the Space Telescope Science Institute, working in partnership with Caltech/IPAC, Center for Astrophysics | Harvard & Smithsonian, and the Jet Propulsion Laboratory.
TAGS: JWST, James Webb Space Telescope, electromagnetic spectrum, exoplanets, universe, solar system, big bang, cosmology, astronomy, star formation, galaxy, galaxies, telescope, life, technology, MIRI, Mars, Engineering, Teaching, Education, Classroom, Science, Universe of Learning