Lesson .
.Pi in the Sky 8
Overview
In the eighth installment of the "Pi in the Sky" illustrated problem set, students use the mathematical constant pi to solve real-world science and engineering problems. Students will use pi to collect samples from an asteroid, fly a helicopter on Mars for the first time, find efficient ways to talk with distant spacecraft, and study the forces behind Earth's beautiful auroras.
Materials
- Pi in the Sky 8 poster – Download PDF
- Pi in the Sky 8 handouts – Download PDF
- Pi in the Sky 8 answer key – Download PDF
- Pi in the Sky 8 answer handouts – Download PDF (also available as a text-only doc)
- "Pi in the Sky" series slideshow (mobile, tablet and screen-reader friendly)
Background
Captured on Oct. 20, 2020, during the OSIRIS-REx mission’s Touch-And-Go (TAG) sample collection event, this series of images shows the SamCam imager’s field of view as the NASA spacecraft approached and touched asteroid Bennu’s surface. Image credit: NASA/Goddard/University of Arizona | › Full image and caption
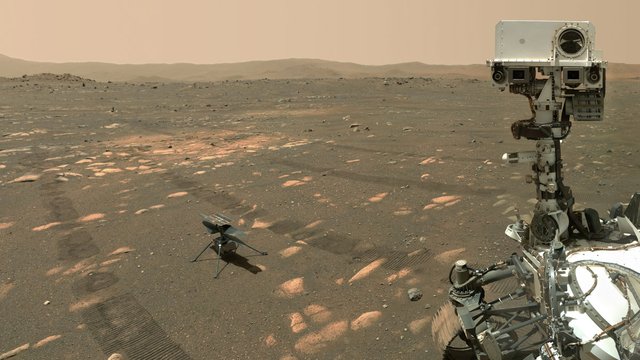
This artist's concept shows what Deep Space Station-23, a new antenna dish capable of supporting both radio wave and laser communications, will look like when completed at the Deep Space Network's Goldstone, California, complex. Image credit: NASA/JPL-Caltech |
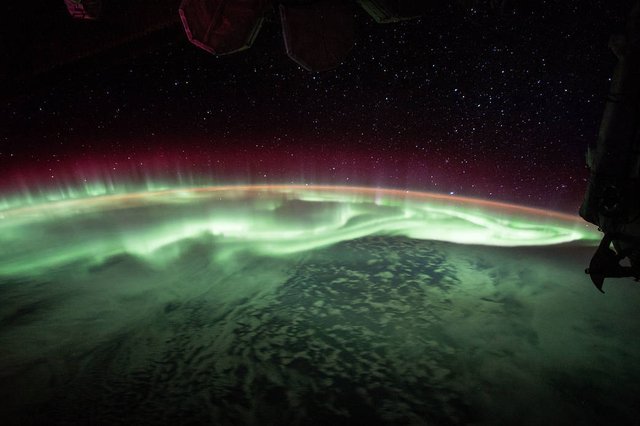
Sample Science
NASA’s OSIRIS-REx mission has flown to an asteroid and collected a sample of surface material to bring back to Earth. (It will arrive back at Earth in 2023.) The mission is designed to help scientists understand how planets form and add to what we know about near-Earth asteroids, like the one visited by OSIRIS-REx, asteroid Bennu. Launched in 2016, OSIRIS-REx began orbiting Bennu in 2018 and successfully performed its maneuver to retrieve a sample on October 20, 2020. In the Sample Science problem, students use pi to determine how much of the spacecraft's sample-collection device need to make contact with the surface of Bennu to meet mission requirements for success.
Whirling Wonder
Joining the Perseverance rover on Mars is the first helicopter designed to fly on another planet. Named Ingenuity, the helicopter is a technology demonstration, meaning it's a test to see if a similar device could be used for a future Mars mission. To achieve the first powered flight on another planet, Ingenuity must spin its blades at a rapid rate to generate lift in Mars’ thin atmosphere. In Whirling Wonder, students use pi to compare the spin rate of Ingenuity’s blades to those of a typical helicopter on Earth.
Signal Solution
NASA uses radio signals to communicate with spacecraft across the solar system and in interstellar space. As more and more data flows between Earth and these distant spacecraft, NASA needs new technologies to improve how quickly data can be received. One such technology in development is Deep Space Optical Communications, which will use near-infrared light instead of radio waves to transmit data. Near-infrared light, with its higher frequency than radio waves, allows for more data to be transmitted per second. In Signal Solution, students can compare the efficiency of optical communication with radio communication, using pi to crunch the numbers.
Force Field
Earth’s magnetic field extends from within the planet and into space, and it serves as a protective shield, blocking charged particles from the Sun. Known as the solar wind, these charged particles of helium and hydrogen race from the Sun at hundreds of miles per second. When they reach Earth, they would bombard our planet and orbiting satellites were it not for the magnetic field. Instead, they are deflected, though some particles become trapped by the field and are directed and concentrated toward the poles, where they interact with the atmosphere, creating auroras. Knowing how Earth’s magnetic field shifts and how particles interact with the field can help keep satellites in safe orbits. In Force Field, students use pi to calculate how much force a hydrogen ion would experience at different points along Earth’s magnetic field.
Procedures
Sample Science
NASA’s OSIRIS-REx mission was designed to travel to an asteroid called Bennu and bring a small sample back to Earth for further study. To achieve its mission, the spacecraft needed to make contact with 26 cm2 of asteroid Bennu’s surface and collect millimeter-size particles using its "contact-pad samplers." These are 1.5-centimeter diameter circular pads of Velcro-like stainless steel. There are 24 pads on the mechanism designed to collect the samples.
How many pads needed to make contact with Bennu's surface to meet the mission requirement?
If all 24 pads contacted Bennu, how much asteroid surface area would the contact pads sample?
Whirling Wonder
Joining the Perseverance rover on Mars is a small helicopter named Ingenuity. With twin counter-rotating blades spanning 1.2 meters, Ingenuity is a test of new technology and is designed to achieve the first powered flight on another world.
Despite Mars having less gravity than Earth, the atmosphere on the Red Planet is much thinner than it is here on our home planet. This makes it challenging to lift off the ground on Mars. To generate enough lift for Ingenuity, engineers determined that the helicopter's blades needed to rotate at approximately 250 radians per second on Mars.
How fast – in rotations per minute – do Ingenuity’s blades spin?
How does that compare to a typical helicopter on Earth with blades that spin at 500 rotations per minute?
Signal Solution
As more and more data are collected and transmitted through space, NASA needs new technologies to communicate faster and more efficiently with its spacecraft. One such technology is called Deep Space Optical Communications, or DSOC, which uses near-infrared light instead of radio waves to transmit a signal. This allows us to use a higher frequency (shorter wavelength), so more data can be transmitted per second.
The twin Voyager spacecraft launched in 1977 use a 12.5 Watt transmitter paired with a parabolic reflector that creates a circular radio signal with a diameter roughly 0.5 degrees wide. A DSOC system would use a 4 Watt transmitter on a flight laser transceiver, producing a light signal with a diameter of 0.0009 degrees.
If Voyager and a DSOC-equipped spacecraft were both placed 124 AU from Earth (where 1 AU = 150,000,000 km) what fraction of each original wattage would be received by a 70m antenna back on Earth?
By what factor is DSOC more effective?
Force Field
Every day, Earth is showered in radiation from the Sun. The Sun also emits charged particles almost entirely in the form of ionized hydrogen and helium. These ions travel at speeds of about 400 km per second but rarely reach Earth's surface. That’s because they are deflected by Earth’s magnetic field due to the Lorentz force, given by the equation: F = qvBsinθ where F = force (N) q = charge of the particle in coulombs (C) v = velocity of the particle in meters per second (m/s) B = the magnetic flux density of Earth’s magnetic field in teslas (T) θ in radians.
The charged particles can't cross Earth's magnetic field, so they follow it to Earth's North and South poles. The resulting concentration of charged particles is what creates auroras.
If Earth’s magnetic flux density is 60µT, what force would a hydrogen ion observe at π/4 radians from the equator? What about at the North Pole (π/2 radians)?
Does the relative magnetic field agree or disagree with what you’d expect about the location of auroras?
Assessment
Extensions
Pi Day Resources
Plus, join the conversation using the hashtag #NASAPiDayChallenge on Facebook, Twitter, and Instagram.
Related Lessons for Educators
Related Activities for Students
Multimedia
- Infographic: Solar Wind
Facts and Figures
Missions and Instruments
Websites
Lesson Last Updated: Oct. 11, 2024