Classroom Activity
Modeling Weather Patterns and the Coriolis Effect
Overview
In this activity, students learn how pressure differences cause air to move from high pressure to low pressure areas, and how the Coriolis effect deflects wind in a predictable pattern.
Materials
Management
- Consider doing only Part 1, only Part 2, or both, depending on student abilities.
- A globe or digital representation of Earth can help students visualize the rotational direction of Earth as seen from the north and south poles.
Background
NASA has been launching balloons from locations around the world for technology development, scientific research, and education purposes since 1983. Balloon missions are not only cheaper to operate than space missions, but they also take less time to plan and develop than space missions. Because of this, they can use higher-risk new or state-of-the-art technologies that haven't yet flown in space.
Antarctica provides a unique environment that scientists can use for balloon launches to study space. The 24 hours of sunlight per day provided by the Antarctic spring and summer allows for solar powered balloon missions to generate power around the clock.
Antarctica is also home to a seasonal weather phenomenon known as an anticyclone – an upper-atmosphere counter-clockwise wind flow. During the late Antarctic spring, a high pressure system forms over the south pole. The difference in air pressure between the south pole (high air pressure) and the air pressure area around the lower southern latitudes (low air pressure) creates a wind that moves outward from the pole toward the low pressure areas. As Earth rotates clockwise as seen from above the south pole, that wind is deflected to the left in a circular pattern. The air current circles the South Pole in a counter-clockwise direction about every two weeks and allows for long balloon flights of missions that can be recovered and potentially reflown.

The red line shows the flight path of STO-1 around Antarctica. The payload launched from the Long Duration Balloon facility in Antarctica on January 15, 2012, and floated in a nearly perfect circle counter-clockwise around the continent for 12 days until it was cut down and recovered about 60 miles (100 kilometers) from the launch site. Image credit: NASA | + Expand image
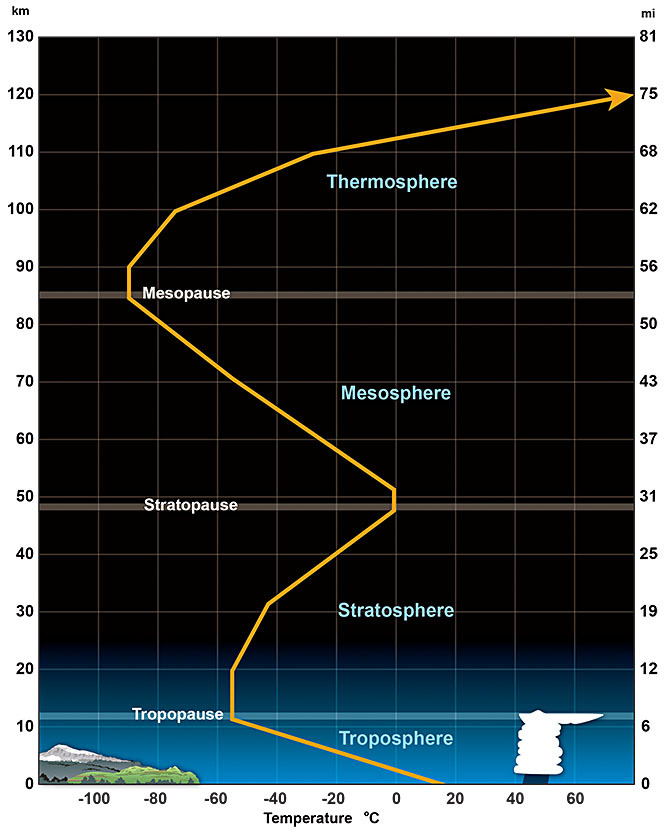
Average temperature profile for the lower layers of the atmosphere. Image credit: NOAA | + Expand image
Sending balloons into the stratosphere offers another advantage. At 130,000 feet (40,000 meters), balloon-based missions are above 99% of the gas in the atmosphere, allowing them to make space-like observations while aloft. Additionally, this layer of the atmosphere warms as altitude increases, making the top of the stratosphere warmer than the bottom. The consistent progression from colder air at the bottom to warmer air at the top prevents mixing and air turbulence, making the air very stable and providing a great place to observe space. You may have noticed this stability if you’ve seen a flat-topped anvil-shaped storm cloud. That flat top is the cloud reaching the bottom of the stratosphere, where the stable air prevents the cloud from rising further.
In December 2024, NASA plans to launch a stratospheric balloon-borne radio-telescope called ASTHROS. Lifted by a 400-foot (120-meter) diameter helium balloon, the mission will spend three to four weeks floating about 130,000 feet above Antarctica. At this altitude, ASTHROS will observe star-forming regions of space in far-infrared light to provide new information about stellar feedback, a process in which stars either accelerate or decelerate the formation of new stars in their galaxy.
Procedures
Part 1
- Tell students they’re going to create a model that shows the movement of wind and how Earth’s rotation affects the direction wind travels. Blow up a balloon and pinch it closed, but don’t tie it off. Ask students what will happen if you hold the balloon, but unpinch the opening. After taking student responses and letting the air out of the balloon, ask students to describe what happened and why.
If necessary, explain that there is more air in the balloon than in the same volume of space outside the balloon, making the air pressure inside the balloon higher than outside. The Pressure Gradient Force causes the high pressure air to move toward the lower pressure area outside of the balloon. This is the same reason air flows out of a tire when the valve is pressed. Note: some students may comment on the elastic nature of the balloon squeezing the air, which is also correct, but simply adds to the high pressure of the air in the balloon. - Ask students to think about the wind and, based on what they just heard about high and low pressure, describe what they think causes wind. If necessary, explain that pockets of air in the atmosphere can have different pressures and wind is caused when air moves from a high pressure area to a low pressure area. Discuss that there is wind that moves from high pressure to low pressure areas at Earth’s surface, called surface winds, and wind that moves from high to low pressure areas high in the atmosphere. Explain that they will be focusing on wind high in the atmosphere.
- Show students the north pole on a globe or the Student Worksheet. Ask them which way Earth rotates from the perspective above the north pole and to explain how they know. Students should state it moves counter-clockwise and explain their reasoning. Repeat the process for the south pole, where Earth rotates clockwise, as seen from above the south pole.
- In pairs or in groups of three, have students complete Part 1 of the student worksheet. Then regroup and discuss the students’ findings.
Part 2
- Have students think about what happens when a helium balloon is released and ask them to explain why that happens. If necessary, explain that the balloon rises because helium is less dense than the surrounding air. If they didn’t mention it, remind them that the balloon will eventually pop. As the balloon rises, the surrounding air pressure gets lower and the growing difference in air pressure between the inside of the balloon and outside of the balloon causes it to expand, finally popping when the balloon can’t stretch any further.
Students may have experienced this drop in pressure on a small scale when they’ve ridden in a car up a large hill, taken an elevator up a very tall building, or flown in an airplane. The feeling of needing to “pop” your ears is the difference in air pressure inside your ears and the outside air. - Tell students that scientific balloons, like the one carrying the ASTHROS mission to high altitudes, rise at a predictable rate. They will complete Part 2 of the student worksheet to graph the ASTHROS balloon’s ascent over time and predict what will happen to the shape of the balloon as it rises.
Discussion
- What direction do winds flow from the south pole and why?
- Counter-clockwise, as seen from above, because they get deflected to the left as they move from high pressure to low pressure.
- Share that this deflection is called the Coriolis effect.
- Where do the winds stop moving away from the pole, and why?
- They stop moving away when they reach the lower pressure area because there is no longer the pressure difference.
- NASA can take advantage of this weather pattern by launching scientific balloons high into the atmosphere from the south pole. What advantage can you think of?
- The balloons stay above the south pole instead of floating away over the ocean.
Assessment
- Student worksheets should show arrow lines moving from the high pressure zone to the low pressure zone on page 3.
- Student worksheets should show curved arrow lines moving from the high pressure zone to the low pressure zone on the map on page 4.
- Student worksheets should show a graph that accurately displays an ascent rate of 1,000 feet per minute.