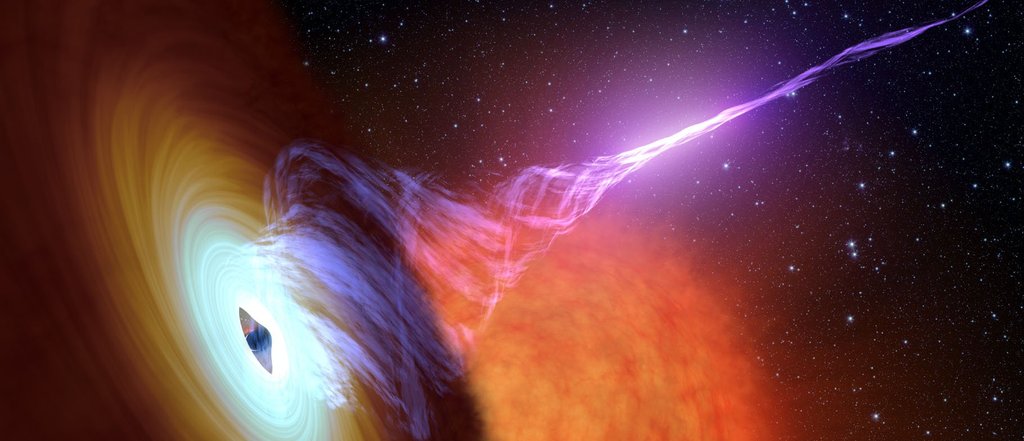
Science
Cosmology
Cosmology is the study of the origin, structure, and evolution of the Universe. We now know that our Universe began 13.8 billion years ago in a hot, dense state we call the Big Bang, then underwent a brief period of incredibly rapid, exponential expansion called inflation, then slowed down to a much slower expansion that has lasted ever since. Only 4.9% of the total mass-energy density of the Universe is in the kind of matter that we are made of, about 26.5% is in “dark” matter, so-called because it interacts only gravitationally with other matter, and is therefore invisible at all wavelengths, and 68.5% is in something we call “dark energy”.
Gravity, acting on quantum fluctuations in the density of matter and energy at the end of inflation, led to the formation of the grand structures of galaxies and clusters of galaxies that we see today. Most of this knowledge has come from observations of the cosmic microwave background (CMB) radiation, the oldest light in the Universe, coming to us from just 470,000 years after the Big Bang. JPL was involved in the first space mission to measure the CMB, the Cosmic Background Explorer, led US participation in the ESA Planck mission, the third generation space CMB mission, numerous balloon and ground-based experiment such as Boomerang, CBI, QUIET, Bicep, and Bicep/Keck, is participating in the next- generation ground-based experiment CMB-S4, and is leading studies of a possible fourth-generation space experiment. But outstanding questions remain. What is the dark matter? What is dark energy? What is the physics of inflation? These questions will be addressed by future missions including Euclid, the Roman Space Observatory, and CMB polarization missions, in which JPL is playing important roles.
Stars and galaxies form by the gravitational collapse of giant clouds of gas – mostly hydrogen and helium. The first stars formed maybe a few hundred million years after the Big Bang, and the peak of star formation occurred after 3 or 4 billion years. Galaxies are giant collections of stars and dark matter. Less than 10% of all the baryons (protons, neutrons, electrons — the kind of matter stars and we are made of) wind up in stars.
Where are the rest of them, and what limits the infall of baryons into galaxies, and the collapse of clouds of baryons, so that all of the baryons don’t wind up in stars? How do things change over time?
The James Webb Space Telescope will be the next major space observatory to address these questions. Its mid-infrared instrument MIRI, key parts of which came from JPL, will play an important role. Ideas for other space missions that will observe in other parts of the spectrum from X-rays to submillimeter wavelengths are in development.
Exoplanets
Since the first tentative detection of a planet around another star in 1988, the study of exoplanets has grown from speculation to one of the most active in all of astrophysics. More than 4,000 exoplanets have been identified, and the number is increasing rapidly. But the study of exoplanets is still in its infancy. How do planets form and evolve? How do planets and their stars interact? What are planets like, physically, chemically, and dynamically? How many habitable planets are there? And the biggest question of all, are we alone?
Most stars have planets, often many. There are many ways to find exoplanets, including indirect ones such as radial velocity and astrometric measurements of host starts, and more direct ones such as microlensing, and transit photometry. None is effective over the full range of stars and planets. Some can be done from the ground, some require space. The masses of planets can be determined by radial velocity measurements and astrometry. Beyond that, reflection or transmission spectra over a wide range of wavelengths of planetary surfaces or atmospheres are essential for characterization and in the search for evidence of life elsewhere in the Universe. Since the stars that they orbit are millions to billlions of times brighter than the planets, such measurements are difficult. Spectra of Earth-like planets around Sun-like stars are not possible from the ground, even with 30 and 40-m telescopes.
JPL has built the first radial-velocity spectrograph fed by an adaptive optics system and coupling the light of a star through a single-mode optical fiber, and is involved in multiple radial velocity technologies and science instruments under construction. The Coronagraph Instrument (CGI) on the Roman Space Telescope, now under construction at JPL, will be the first ever to reach a contrast of hundreds of millions. JPL led the NASA “decadal mission” study for an even more ambitious mission, HabEX, that would have both a coronagraph and a starshade and achieve contrast ratios of about 10 billion, enabling spectra of Earth-like planets around Sun-like stars.
To learn more about how NASA studies exoplanets/our universe, visit exoplanets.nasa.gov and universe.nasa.gov
Gravitational Waves, Detection, Evolution of Collapsed Objects
The General Theory of Relativity, or GR, predicted over 100 years ago that disturbances in space- time caused by the acceleration of mass would travel through the Universe at the speed of light.
The first observational confirmation of the existence of gravitational waves came from observations in 1974 of two pulsars — anisotropically radiating, spinning neutron stars – orbiting each other in a binary system. The orbital period decreased over time as some of the energy in the system was emitted in the form of gravitational waves, exactly as predicted by GR.
The first direct detection of gravitational waves was made by the LIGO experiment in 2015. The radiation was emitted when two black holes of 36 and 29 times the mass of our Sun coalesced into a single black hole of 62 solar masses. About three solar masses were converted into the energy of the gravitational waves.
Gravitational waves provide a new window on the Universe that will tell us about the merger of compact objects from neutron stars up to billions of solar masses. JPL is participating in the laser interferometer space antenna, LISA, an ESA-led mission scheduled to launch in 2034 that will detect gravitational waves in the frequency range 10^(−4)–1 Hz, compared to LIGO’s 10–100 Hz. JPL is also part of the North American Nanohertz Observatory for Gravitational Waves (NANOGrav), which will detect gravitational waves in the 16^(−9) Hz range through observations of timing changes in pulsars.
Fundamental Physics
The Cold Atom Laboratory, built at JPL, installed in the International Space Station in late 2016, and updated in late 2019, cools atoms of rubidium and potassium to extremely low temperatures in zero-g conditions to test and measure the quantum behavior of matter in ways not possible on the ground.
CAL has demonstrated the first Bose-Einstein condensates in space, achieved record low temperatures, and demonstrated wave-like interference in atoms. These fundamental behaviors may in future be exploited to make measuring instruments of exquisite precision. CAL is the first of what may become a steady progression of physics laboratories in space.
CL#22-0773