is the Story of the Solar System
The principal goal of NASA’s Juno mission is to understand the origin and evolution of Jupiter. Underneath its dense cloud cover, Jupiter safeguards secrets about the fundamental processes and conditions that governed our solar system during its formation. As our primary example of a giant planet, Jupiter can also provide critical knowledge for understanding the planetary systems being discovered around other stars.
With its suite of science instruments, Juno will investigate the existence of a possible solid planetary core, map Jupiter’s intense magnetic field, measure the amount of water and ammonia in the deep atmosphere, and observe the planet’s auroras.
Juno will let us take a giant step forward in our understanding of how giant planets form and the role these titans played in putting together the rest of the solar system.

Jupiter’s Origins and Interior
Theories about solar system formation all begin with the collapse of a giant cloud of gas and dust, or nebula, most of which formed the infant sun, our star. Like the sun, Jupiter is mostly hydrogen and helium, so it must have formed early, capturing most of the material left after our star came to be. How this happened, however, is unclear. Did a massive planetary core form first and capture all that gas gravitationally, or did an unstable region collapse inside the nebula, triggering the planet’s formation? Differences between these scenarios are profound.
Even more importantly, the composition and role of icy planetesimals, or small protoplanets, in planetary formation hangs in the balance — and with them, the origin of Earth and other terrestrial planets. Icy planetesimals likely were the carriers of materials like water and carbon compounds that are the fundamental building blocks of life.
Unlike Earth, Jupiter’s giant mass allowed it to hold onto its original composition, providing us with a way of tracing our solar system’s history. Juno will measure the amount of water and ammonia in Jupiter’s atmosphere and help determine if the planet has a core of heavy elements, constraining models on the origin of this giant planet and thereby the solar system. By mapping Jupiter’s gravitational and magnetic fields, Juno will reveal the planet’s interior structure and measure the mass of the core.
Atmosphere
How deep Jupiter’s colorful zones, belts and other features penetrate is one of the most outstanding fundamental questions about the giant planet. Juno will determine the global structure and motions of the planet’s atmosphere below the cloud tops for the first time, mapping variations in the atmosphere’s composition, temperature, clouds and patterns of movement down to unprecedented depths.

Magnetosphere

Juno will provide the first close look at Jupiter's poles, probing forces in the magnetosphere that connect the giant, fast-rotating planet's exterior with its deep interior. (Background image from Cassini; top inset: Jupiter auroras from the Hubble Space Telescope; bottom inset: Cassini at Saturn) Credit NASA/JPL-Caltech
Deep in Jupiter’s atmosphere, under great pressure, hydrogen gas is squeezed into a fluid known as metallic hydrogen. At these enormous pressures, the hydrogen acts like an electrically conducting metal, which is believed to be the source of the planet’s intense magnetic field. This powerful magnetic environment creates the brightest auroras in our solar system, as charged particles precipitate down into the planet’s atmosphere. Juno will directly sample the charged particles and magnetic fields near Jupiter’s poles for the first time, while simultaneously observing the auroras in ultraviolet light produced by the extraordinary amounts of energy crashing into the polar regions. These investigations will greatly improve our understanding of this remarkable phenomenon, and also of similar magnetic objects, like young stars with their own planetary systems.
Juno Science Objectives
The primary science objectives of the mission are as follows:
Origin
Determine the abundance of water and place an upper limit on the mass of Jupiter’s
possible solid core to decide which theory of the planet’s origin is correct
Interior
Understand Jupiter’s interior structure and how material moves deep within the planet
by mapping its gravitational and magnetic fields
Atmosphere
Map variations in atmospheric composition, temperature, cloud opacity and dynamics
to depths greater than 100 bars at all latitudes.
Magnetosphere
Characterize and explore the three-dimensional structure of Jupiter’s polar
magnetosphere and auroras
The overall goal of the Juno mission is to improve our understanding of the solar system by understanding the origin and evolution of Jupiter. It addresses science objectives central to three NASA Science divisions: Solar System (Planetary), Earth–Sun System (Heliophysics), and Universe (Astrophysics).
Juno’s primary science goal of understanding the formation, evolution, and structure of Jupiter is directly related to the conditions in the early solar system, which led to the formation of our planetary system. The mass of Jupiter’s possible solid core and the abundance of heavy elements in the atmosphere discriminate among models for giant planet formation. Juno constrains the core mass by mapping the gravitational field, and measures through microwave sounding the global abundances of oxygen (water) and nitrogen (ammonia).
Juno reveals the history of Jupiter by mapping the gravitational and magnetic fields with sufficient resolution to constrain Jupiter’s interior structure, the source region of the magnetic field, and the nature of deep convection. By sounding deep into Jupiter’s atmosphere, Juno determines to what depth the belts and zones penetrate. Juno provides the first survey and exploration of the three-dimensional structure of Jupiter’s polar magnetosphere.
Juno's Science Instruments
Microwave Radiometer (MWR)
Juno’s Microwave Radiometer instrument will probe beneath Jupiter’s cloud tops to provide data
on the atmosphere's structure, movement and chemical composition to a depth as great as 1,000
atmospheres — about 342 miles (550 kilometers) below the visible cloud tops. In particular, MWR
will determine the amount of water in the planet's atmosphere.
--Mike Janssen
Juno will use its Microwave Radiometer (MWR) instrument to probe Jupiter's deep atmosphere, revealing new insights about its structure and composition. The instrument will make measurements that enable scientists to determine the amount of water in the planet's atmosphere. This information is the missing key to understanding Jupiter's formation.
To see what’s under the cloud tops, MWR will measure the microwave radiation emitted from inside the planet. The planet emits radiation across the radio, microwave and infrared ranges, but only microwave frequencies can make it out through the thick clouds. The depth from which the radiation can escape depends on frequency, so by measuring different frequencies of microwave radiation, MWR can study different layers of Jupiter’s interior.
Strong radio emission from Jupiter's radiation belts blocks our view of the planet from Earth at the critical microwave frequencies necessary to measure Jupiter's water abundance. Juno avoids this problem by flying close to Jupiter, inside the radiation belts.
MWR consists of six antennas designed to passively sense the microwaves coming from six levels within the clouds. These levels range from the cloud tops, where the pressure is about the same as that on Earth, down to a depth of hundreds of miles, where the pressure is a thousand times greater. The deepest layers (below 100 bars) will reveal Jupiter’s water content, which is key to understanding how Jupiter formed. MWR will also allow us to determine how deeply atmospheric features extend into the planet, including the cloud bands and the Great Red Spot.
All six MWR antennas are located on the sides of Juno’s hexagonal body, with the largest antenna taking up one whole side. Each antenna is connected by a cable to a receiver, which sits in the instrument vault on top of the spacecraft.
The MWR instrument operates during five preselected Juno orbits. During these orbits, Juno is oriented so that the MWR antennas' views sweep across Jupiter directly below the spacecraft. This geometry allows Juno to observe thermal emission from each point along the spacecraft's path over the planet to be observed from multiple angles, helping MWR build up a three- dimensional understanding of how the deep atmosphere is structured.

The largest of Juno's six MWR antennas takes up a full side of the spacecraft.
JPL provided the MWR sub-system components, including the antennas and receivers.
Instrument stats:
Measures microwave brightness temperatures of Jupiter with six passive microwave
antennas, sensitive to wavelengths between 1.3 centimers and 50 centimeters, or
frequencies between 0.6 GHz and 22 GHz
Largest antenna is 5.2 feet (1.6 meters) square.
Location: Two sides of the spacecraft, between the solar arrays
Two largest antennas, which sense the lowest frequencies, are "patch arrays," the next three smaller antennas are "slot arrays," the smallest is a "horn" antenna.
Gravity Science Experiment
Juno's Gravity Science experiment will use the spacecraft's telecommunications system to help us
understand Jupiter’s inner structure, by very precisely mapping the planet's gravitational field.
The Gravity Science experiment will enable Juno to measure Jupiter’s gravitational field and reveal
the planet’s internal structure. Juno will see how the material inside Jupiter churns and flows, helping
to determine whether the planet harbors a dense core at its center.
-- William Folkner
Variations in Jupiter’s inner structure will have tiny effects on its gravitational field, which ever so slightly alters Juno’s orbit. The closer Juno gets to Jupiter, the more pronounced the displacements are. These subtle shifts in Juno’s motion cause equally subtle shifts in the frequency of a radio signal received from and sent back to Earth. Known as the Doppler effect, it’s the same type of frequency shift that happens when the pitch of an ambulance’s siren increases when speeding toward you and decreases when speeding away from you.
To measure these tiny shifts, Juno’s telecommunication system is equipped with a radio transponder that operates in the X band, which are radio signals with a wavelength of three centimeters. The transponder detects signals sent from NASA’s Deep Space Network on Earth and immediately sends a signal in return. The small changes in the signal’s frequency tell us how much Juno has shifted due to variations in Jupiter’s gravity. For added accuracy, the telecommunication system also has a Ka- band translator system, which does a similar job, but at radio wavelengths of one centimeter. One of the antennas of NASA's Deep Space Network located in Goldstone, California, has been fitted to send and receive signals at both radio bands. An instrument called the Advanced Water Vapor Radiometer helps to isolate the signal from interference caused by Earth’s atmosphere.
JPL provided the Juno telecom system. The Italian Space Agency contributed the Ka-band translator system.

The Doppler shift in Juno's radio signal will allow scientists to map variations in Jupiter's gravity field as the spacecraft falls past the planet during each orbit.
Instrument stats:
Frequencies used for transmitting gravity data:X-band and Ka-band
Location: Saucer-shaped high-gain antenna on top of spacecraft and radio transponder within radiation vault
Magnetometer Experiment (MAG)
Juno's magnetometer will visualize Jupiter's magnetic field in 3-D, all around the planet, sensing the
deep interior and watching for changes over time.
Using its magnetometer (MAG), Juno will create an extremely accurate and detailed three-dimensional map of Jupiter’s magnetic field. This unprecedented study will allow us to understand Jupiter’s internal structure and how the magnetic field is generated by the dynamo action inside – the churning of electrically charged material deep below the surface. MAG will also monitor the field for long-term variations, called secular variations, during the entire mission. Measurements of these variations, in combination with the map, will help us determine the depth of the dynamo region. Because Jupiter lacks a rocky crust or continents that complicate the picture as they do at Earth, Juno’s observations could be the most detailed look at a planetary dynamo ever.
-- Jack Connerney
Electrical currents can align themselves with the magnetic field, and these so-called Birkeland currents help drive the brilliant auroras around Jupiter’s poles. To better understand the auroras, MAG will measure these currents.
MAG consists of two flux gate magnetometers, which will measure the strength and direction of the magnetic field lines, and an advanced stellar compass (ASC) -- a system of four star cameras that will monitor the orientation of the magnetometer sensors.
Juno’s other instruments have their own small magnetic fields, and to avoid contamination, the MAG sensors sit as far from the rest of the spacecraft as possible. They are mounted on the magnetometer boom that sticks out from one of Juno’s solar arrays. As an extra precaution, there are two sets of MAG sensors – one 33 feet (10 meters) from the center of the spacecraft and one 39 feet (12 meters) from the center. By comparing measurements from both sensors, mission scientists can remove from the MAG data any contamination due to the spacecraft itself.
NASA's Goddard Space Flight Center provided the Juno magnetometers. The ASC is provided by Danish Technical University.
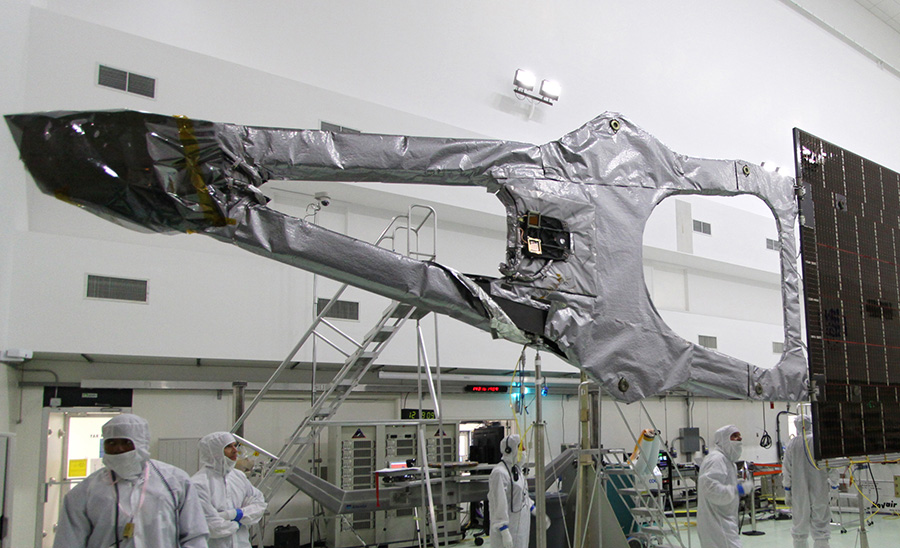
Four star tracker cameras help determine the precise orientation of the magnetometers as they sense Jupiter's magnetic field.
Instrument stats:
Sensors: 2 fluxgate magnetometers, plus four advanced star tracker cameras
Location: Mounted on a boom at the end of one of Juno's solar arrays
Jovian Auroral Distributions Experiment (JADE)
JADE is a set of sensors charged with detecting the electrons and ions that produce Jupiter’s auroras.
The JADE instrument will work with some of Juno’s other instruments to identify the particles and processes that produce Jupiter’s stunning auroras. It will also help create a three-dimensional map of the planet’s magnetosphere.
-- Phil Valek
JADE consists of four sensors that share an electronics box. Three of the sensors will detect electrons in the space surrounding Juno, while the fourth will identify positively charged hydrogen, helium, oxygen and sulfur ions. Most of these ions are ejected from the volcanoes on Jupiter’s moon Io.
When Juno flies directly over Jupiter's auroras, JADE will be able to observe the light show, resolving structures as small as 50 kilometers (30 miles). Considering that the auroras can stretch for tens of thousands of kilometers around the pole, JADE will be able to discern a lot of detail. JADE will also measure the particles that fly to and from Jupiter’s poles, spiraling along as they’re guided by the magnetic field. JADE is provided by SwRI.
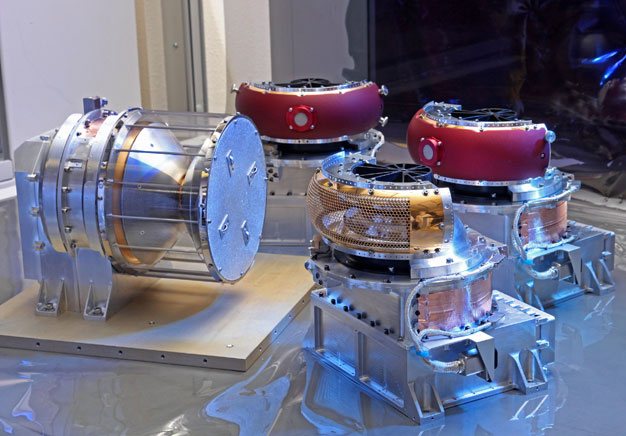
The JADE investigation has two types of sensors to detect the charged particles that create Jupiter's stunning auroras: one for ions and one for electrons.
Instrument stats:
Number of sensors: 4 (3 electron sensors, 1 ion sensor)
Location: upper spacecraft deck
Measures electrons in the energy range from 100 eV to 95 keV
Measures ions in the energy range from 10 eV to 46 keV
Jupiter Energetic-Particle Detector Instrument (JEDI)
JEDI will measure the energetic particles that stream through space and study how they interact with
Jupiter’s magnetic field.
-- Barry Mauk
The Jupiter Energetic Particle Detector Instrument (JEDI) will measure energetic particles that stream through space around Jupiter, studying how they interact with Jupiter’s magnetic field. These electrically charged particles -- consisting of electrons and ions -- follow the influence of the magnetic field. Many of them are channeled by the field toward Jupiter’s poles, where they crash into the atmosphere and create brilliant auroras.

Each of the three JEDI detectors consists of a disk the size and shape of a hockey puck attached to an electronics box.
JEDI will determine the amount of energy these particles carry, their type and the direction in which they’re zipping around Jupiter. Working with Juno’s other instruments designed to study the magnetosphere -- the bubble created by Jupiter’s magnetic field -- JEDI will help us understand how the planet's auroras are produced, as well as the processes by which the magnetic field interacts with charged particles to dump energy into the planet’s atmosphere.
JEDI comprises three identical sensor units each with six ion and six electron views. JEDI works in close coordination with the JADE instrument. JEDI measures the higher-energy charged particles in Jupiter's environment, while JADE measures the lower-energy ones. The instrument also works in coordination with the JADE and Waves instruments to investigate Jupiter’s polar space environment, with a particular focus on the physics of Jupiter’s intense and impressive northern and southern auroral lights.
JEDI uses measurement techniques and technologies demonstrated previously at Jupiter by NASA’s Galileo mission, and is similar to the New Horizons Pluto Energetic Particle Spectrometer Science Investigation (PEPSSI).
JEDI is provided by APL.
Instrument stats:
Number of sensors: 3
Location: Upper spacecraft deck
Measures electrons in the energy range of 25 keV to 800 keV
Measures ion in the energy range of 10 keV to 8000 keV
Jovian Infrared Auroral Mapper (JIRAM)
JIRAM will provide a visual and thermal (infrared) view of Jupiter’s aurora.
JIRAM will study Jupiter's atmosphere in and around Jupiter's auroras, providing new insights about the interactions between the auroras, the magnetic field and the magnetosphere. JIRAM will be able to probe the atmosphere down to 30 to 45 miles (50 to 70 kilometers) below the cloud tops, where the pressure is five to seven times greater than on Earth at sea level.
-- Alberto Adriani
JIRAM consists of a camera and a spectrometer, which splits light into its component wavelengths, like a prism. The camera will take pictures in infrared light, which is heat radiation with wavelengths of two to five microns (millionths of a meter) -- three to seven times longer than visible wavelengths. In particular, the instrument will snap photos of auroras at a wavelength of 3.4 microns -- the wavelength of light emitted by excited hydrogen ions in the polar regions. Methane in the atmosphere absorbs light at this same wavelength, darkening the atmosphere behind the auroras. In front of a darkened background, the auroras stand out even more brightly.

JIRAM is an infrared spectrometer designed to observe Jupiter's auroras and atmosphere from very close to the planet.
The instrument will also try to learn about the structure and origin of voids in Jupiter’s atmosphere called hot spots. These spots -- like the one the Galileo probe happened to have dropped into in 1995 -- are windows into the depths of Jupiter’s atmosphere. By measuring the heat radiating from Jupiter’s atmosphere, JIRAM can determine how water-containing clouds circulate under the surface. It turns out that this sort of motion, called convection, in which hot gas rises and cool gas sinks, reveals the amount of water in these clouds. Certain gases in the atmosphere -- methane, water, ammonia and phosphine, in particular -- absorb particular wavelengths of infrared light. When the spectrometer measures the infrared rainbow emitted by Jupiter, the wavelengths of light absorbed by those gases will be reduced by an amount that indicates the chemical composition of the atmosphere.
JIRAM was developed by the Italian National Institute for Astrophysics and funded by the Italian Space Agency.
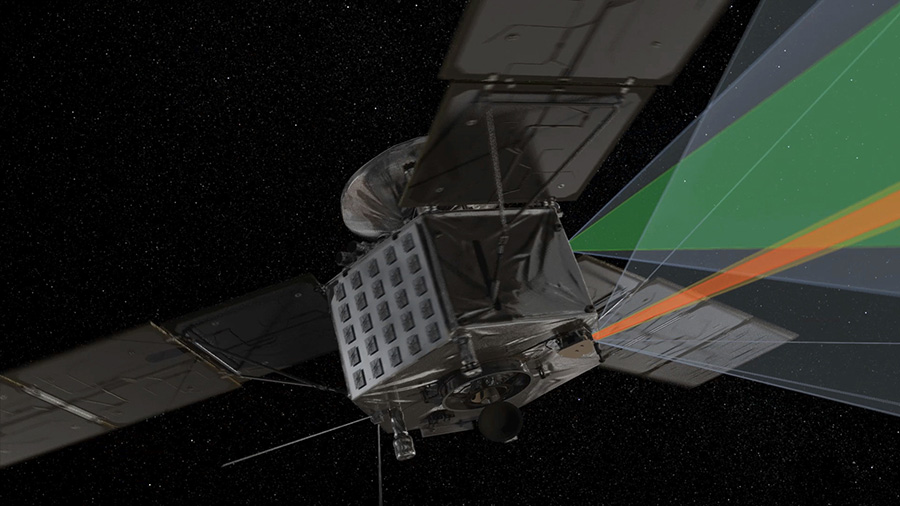
The JIRAM instrument is mounted to the aft deck of the Juno spacecraft and looks in the same direction as the other optical instruments, UVS and JunoCam.
Instrument stats:
Spectral range: Sensitive to infrared wavelengths between 2 and 5 microns.
Spectral resolution: 9 nanometers
Location: Mounted to the aft deck of the spacecraft
Operating temperature: 100 Kelvin or lower
Ultraviolet Imaging Spectrograph (UVS)
UVS will see Jupiter’s auroras in UV, which helps us understand Jupiter’s upper atmosphere and the
particles that slam into it, creating the greatest light show in the solar system.
UVS will take pictures of Jupiter’s auroras in ultraviolet light. Working with Juno’s JADE and JEDI instruments, which measure the particles that create the auroras, UVS will help us understand the relationship between the auroras, the particles that collide with Jupiter's atmosphere to create them, and the planet's magnetosphere as a whole.
-- Randy Gladstone
NASA’s Hubble Space Telescope has taken impressive images of Jupiter’s auroras, but Juno will get an even better view -- looking directly down on them over the north and south poles. UVS includes a scan mirror for targeting specific auroral features. The instrument is sensitive to both extreme and far-ultraviolet light, within a wavelength range of about 70 to 200 nanometers. In comparison, visible light has wavelengths that range from 400 to 700 nanometers.
Southwest Research Institute (SwRI) provided the UVS instrument. CSL/BELSPO (Belgium) contributed the scan mirror.

The UVS instrument observes Jupiter's auroras in ultraviolet light, breaking up the light into its component wavelengths to reveal processes that power the planet's brilliant UV light show.
Instrument stats:
Wavelength range: 68 to 210 nanometers
Spectral resolution: 0.6 to 1.2 nanometers
Location: Mounted to side of spacecraft, between solar arrays
Waves
The Waves instrument will measure radio and plasma waves in Jupiter’s magnetosphere, helping us
understand the interactions between the planet's magnetic field, atmosphere and magnetosphere.
Waves will also pay particular attention to activity associated with auroras.
-- Bill Kurth
Jupiter's magnetosphere, an enormous bubble created by the planet's magnetic field, traps plasma, an electrically charged gas. Activity within this plasma, which fills the magnetosphere, triggers waves that only an instrument like Waves can detect.

Waves consists of two sensors that monitor radio and plasma waves in echoing through Jupiter's magnetosphere.
Because plasma conducts electricity, it behaves like a giant circuit, connecting one region with another. Activity on one end of the magnetosphere can therefore be felt somewhere else, allowing Juno to monitor processes occurring in this entire, giant region of space around Jupiter. Radio and plasma waves move through the space around all of the giant, outer planets, and previous missions have been equipped with similar instruments.
Juno's Waves instrument consists of two sensors; one detects the electric component of radio and plasma waves, while the other is sensitive to just the magnetic component of plasma waves. The first sensor, called an electric dipole antenna, is a V-shaped antenna, four meters from tip to tip -- similar to the rabbit-ear antennas that were once common on TVs. The magnetic antenna -- called a magnetic search coil -- consists of a coil of fine wire wrapped 10,000 times around a 6-inch-long (15-centimeter) core. The search coil measures magnetic fluctuations in the audio frequency range.
The University of Iowa provides the instrument.

This artist's rendering shows Juno above Jupiter's north pole, with the auroras glowing brightly. Jupiter's magnetic field surrounds the planet. A radio wave from the auroras is shown traveling past the spacecraft, where it is intercepted by the Waves investigation, whose sensors are highlighted in bright green.
Instrument stats:
Sensors: 2 (electric dipole antenna and magnetic search coil)
Frequency range:
50 Hz (near the bottom of the audio frequency range) to ~40 MHz (the limit of Jupiter's radio
emissions)
Location: Mounted to the aft deck of the spacecraft
JunoCam
JunoCam is Juno's color imaging camera, which will provide close-up views of Jupiter's poles for the first time.
-- Michael Ravine
Juno’s color, visible-light camera, called JunoCam, is designed to capture remarkable pictures of Jupiter’s cloud tops. As Juno’s eyes, it will provide a wide view, helping to provide context for the spacecraft’s other instruments. JunoCam was included on the spacecraft specifically for purposes of public engagement; although its images will be helpful to the science team, it is not considered one of the mission's science instruments.
Juno rotates twice per minute, so JunoCam's images would be smeared if it were to try to take a complete picture at once. Instead, it is a "push-broom imager," taking thin strips of an image at the same rate that the spacecraft spins. JunoCam then stitches the strips together to form the full picture.
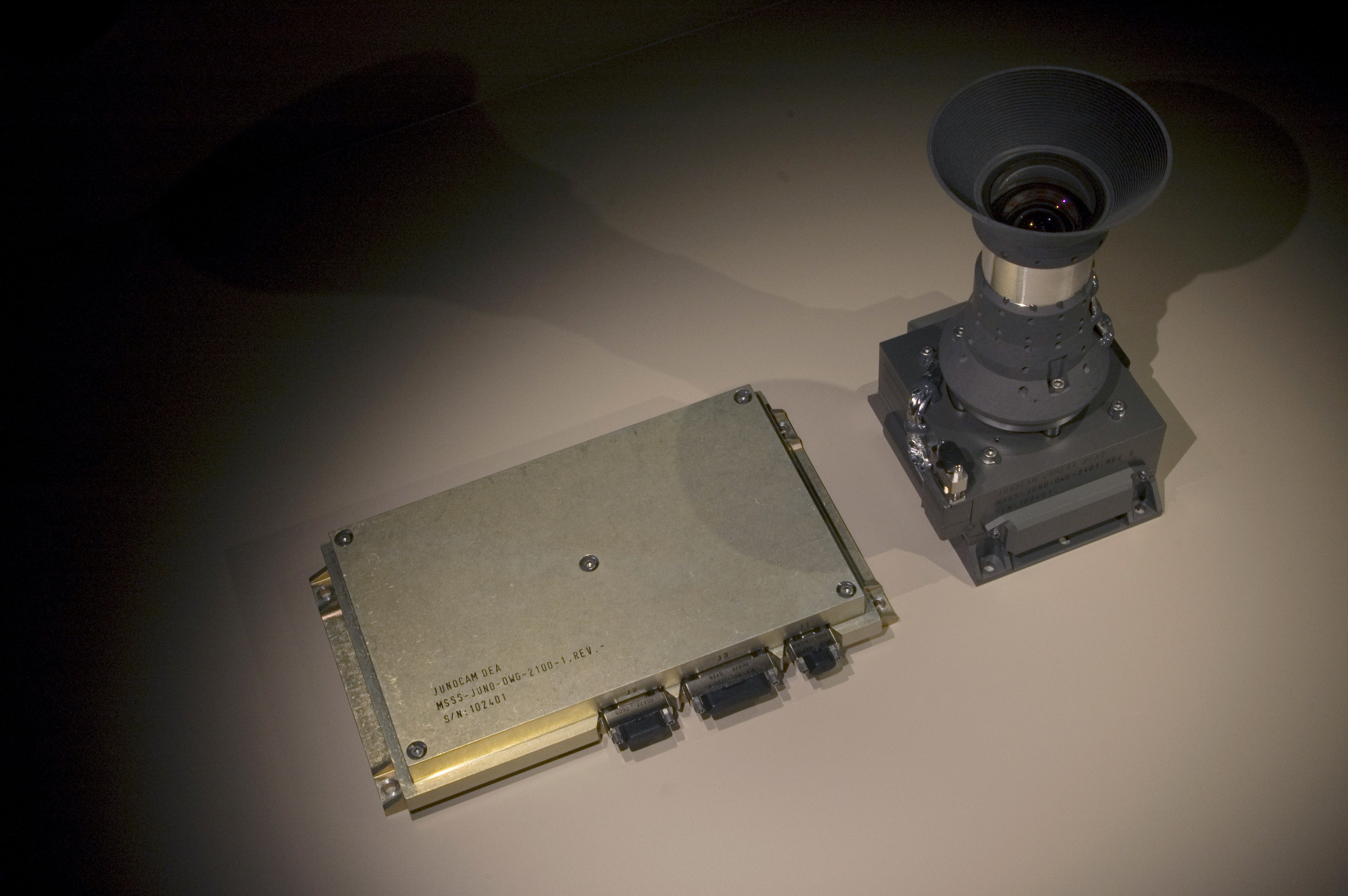
JunoCam consists of a camera head and an electronics box (the box is housed in Juno's protective radiation vault).
JunoCam takes images mainly during closest approach – about 3,100 miles (5,000 kilometers) above the cloud tops – when it has the best-possible vantage point. Taking pictures with a resolution of up to 25 kilometers (16 miles) per pixel, the wide-angle camera will provide high-quality views of Jupiter’s atmosphere. These images will be made available on the Juno mission website for members of the public to process into color views. The public will also help choose targets for JunoCam to image, and members of the amateur astronomy community will provide maps to help in image planning.
The Juno team expects that high-energy particles surrounding Jupiter will eventually damage JunoCam’s electronics to a point where the instrument will have to be shut down permanently. The camera is designed to last for at least seven orbits – enough time to take many pictures.
JunoCam’s hardware is based on a descent camera, called MARDI, that was developed for NASA's Curiosity Mars rover. Some of its software was originally developed for NASA’s Mars Odyssey and Mars Reconnaissance Orbiter spacecraft. JunoCam is provided by Malin Space Science Systems.
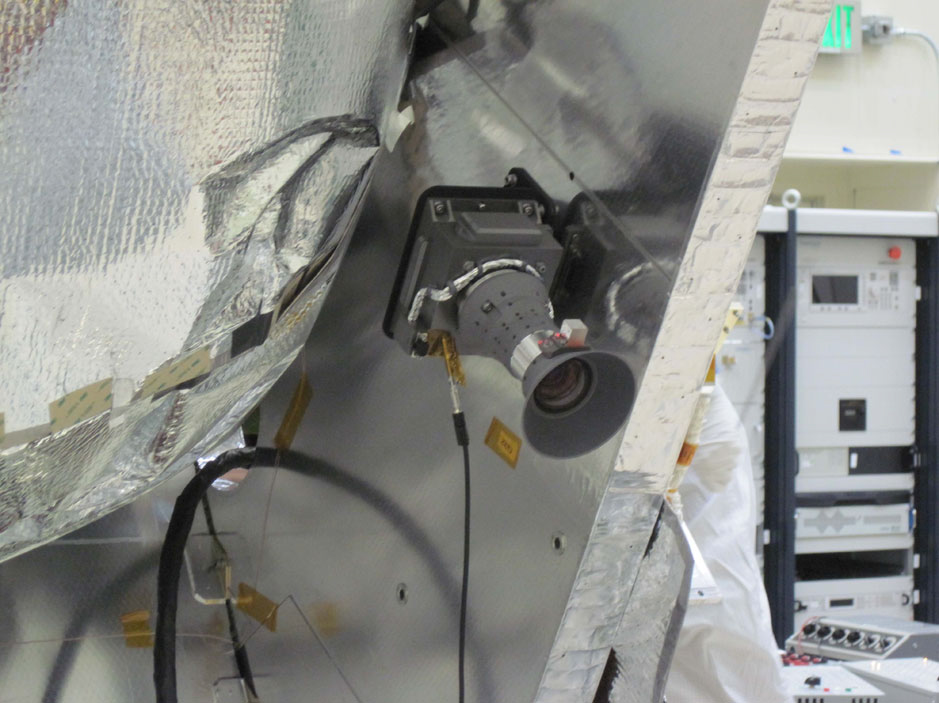
JunoCam is shown mounted to the spacecraft, prior to launch.
Instrument stats:
Image field of view: 58 degrees; 0.7 mrad/pixel
Spectral range: 400-900 nanometers
Spectral filters: 3 RGB color, 1 methane [878-899 nanometers])
Location: Side of spacecraft
Image size: 1600 x 4800 pixel 3-color image;
800 x 2400 pixel
methane-band image
Juno Science Team
Scott Bolton, principal investigator, Southwest Research Institute, San Antonio
John "Jack" Connerney, deputy principal investigator, Goddard Space Flight Center, Greenbelt, Maryland
Steve Levin, project scientist, NASA’s Jet Propulsion Laboratory, Pasadena, California
Instrument Leads
Michael Janssen, MWR Instrument Lead, Jet Propulsion Laboratory, Pasadena, California
John Connerney, MAG Instrument Lead, Goddard Space Flight Center, Greenbelt, Maryland
Phil Valek, JADE Instrument Lead, Southwest Research Institute, San Antonio
William Folkner, Gravity Science Investigation Lead, Jet Propulsion Laboratory, Pasadena, California
Barry Mauk, JEDI Instrument Lead, Johns Hopkins University/Applied Physics Laboratory, Laurel, Maryland
William Kurth, Waves Instrument Lead, University of Iowa, Iowa City
Randy Gladstone, UVS Instrument Lead, Southwest Research Institute, San Antonio
Michael Ravine, JunoCam Instrument Lead, Malin Space Science Systems, San Diego
Alberto Adriani, JIRAM Instrument Lead, Italian Space Agency, Rome
Co-Investigators
Michael Allison | Goddard Space Flight Center, Greenbelt, Maryland |
John Anderson | Jet Propulsion Laboratory, Pasadena, California |
Sushil Atreya | University of Michigan, Ann Arbor |
Fran Bagenal | University of Colorado, Boulder |
Michel Blanc | L’Observatoire Midi Pyrénées, Toulouse, France |
Jeremy Bloxham | Harvard University, Cambridge, Massachusetts |
Shannon Brown | Jet Propulsion Laboratory, Pasadena, California |
Stanley Cowley | University of Leicester, United Kingdom |
Eric DeJong | Jet Propulsion Laboratory, Pasadena, California |
Daniel Gautier | LESIA Observatoire de Paris, France |
Eric Gerard | Université de Liège, Belgium |
Denis Grodent | Université de Liège, Belgium |
Tristan Guillot | Observatoire de la Cote d’Azur, Nice, France |
Samuel Gulkis | Jet Propulsion Laboratory, Pasadena, California |
Candice Hansen | Planetary Science Institute, Tucson, Arizona |
George Hospardarsky | University of Iowa, Iowa City |
William Hubbard | University of Arizona, Tucson |
Luciano Iess | Università La Sapienza, Rome, Italy |
Andrew Ingersoll | California Institute of Technology, Pasadena |
John Jorgensen | Technical University of Denmark |
Philip Louarn | L’Observatoire Midi Pyrénées, Toulouse, France |
Jonathan Lunine | University of Arizona, Tucson |
Glenn Orton | Jet Propulsion Laboratory, Pasadena, California |
Toby Owen | University of Hawaii, Honolulu |
Chris Paranicas | Applied Physics Lab, Laurel, Maryland |
Mike Ravine | Malin Space Science Systems, San Diego |
Ed Smith | Jet Propulsion Laboratory, Pasadena, California |
Paul Steffes | Georgia Tech, Atlanta |
David Stevenson | California Institute of Technology, Pasadena |
Ed Stone | California Institute of Technology, Pasadena |
Richard Thorne | University of California Los Angeles |
Hunter Waite | Southwest Research Institute, San Antonio |